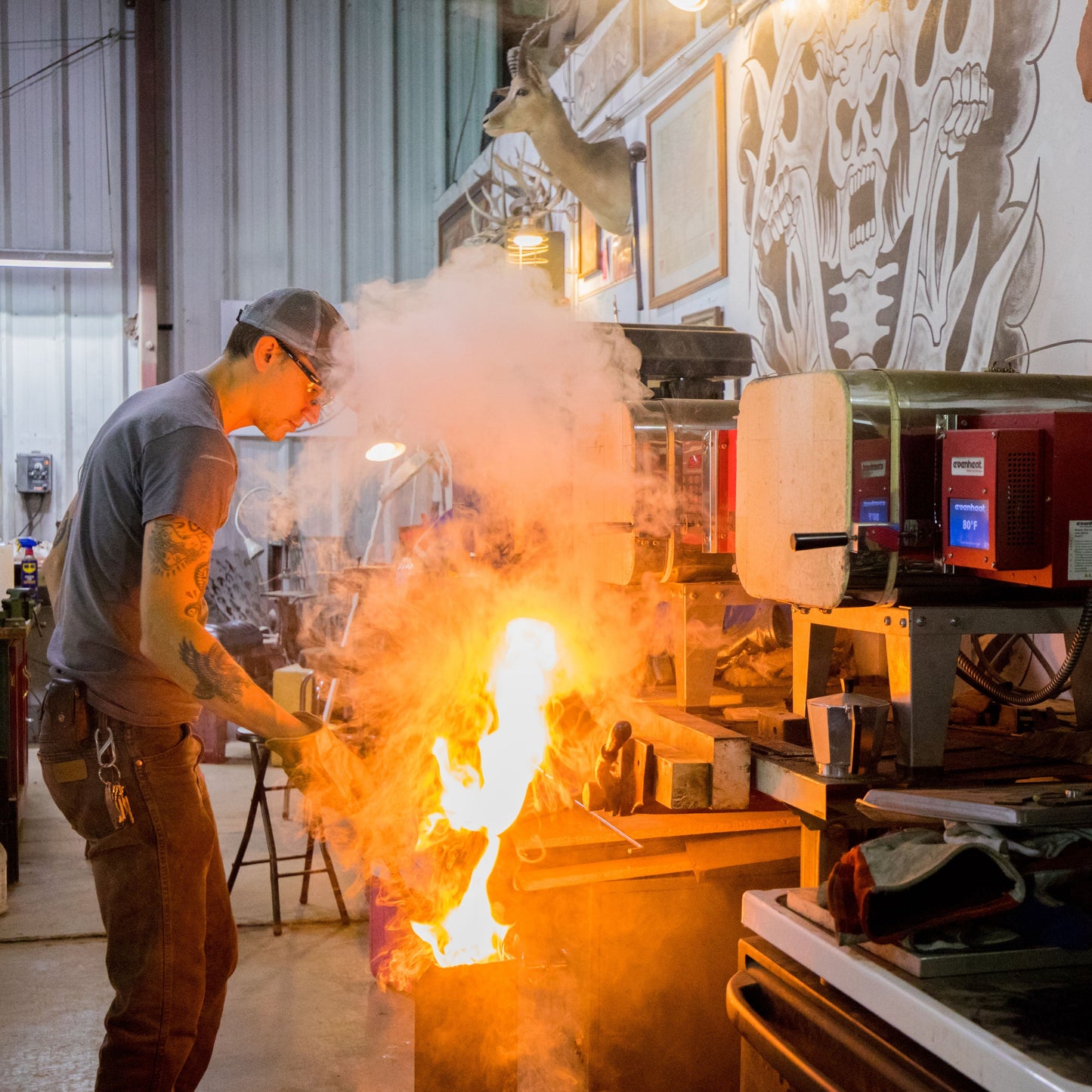
Chaos is clearly the natural state of my knife shop. There is a constant battle against clutter, dust, spills, and general disarray in the shop that I feel like I am always losing. Every day that I sweep the floor, I get a sizable pile of dirt and trash. How does this happen? No one is making the floor dirty or throwing trash down intentionally. The bearings on the grinder wheels regularly go bad and the aluminum wheels themselves wear down. Motors burn out, hammer handles break or get loose, bits and blades seem to last for only minutes before dulling to uselessness. There are mountains of worn out grinding belts laying around and piles of hand sanding paper. My clothes and boots look nice the day I buy them and are instantly worn with a patina of holes, stains and little hard spots where epoxy dried into the fabric.
It appears that there is a high level of entropy in a knife shop. Entropy is the potential amount of energy or change in any given system. In the realm of thermodynamics, entropy is related to heat in that systems with more heat tend to change more rapidly as the potential energy is more easily released. Colder systems tend to slow down the movement of atoms and therefore lock potential energy in place for longer. Water shows this most clearly. When water is very cold, it has a stable form with very low entropy - ice. As it heats up, water changes to a less stable liquid form and then of course steam is the hottest and least stable, making prediction and control more difficult.
The term Entropy is usually used to refer to the tendency of systems to decline into disorder or chaos. So in this case, knife shops are entropic systems. The more stuff in the shop in the form of tools and materials - and the hotter it is, the more potential for change in the direction of disorder. Although high ambient temperatures in summer definitely make things more difficult, most of our heat problems come as the result of friction. Those bearings and motors are degrading as a result of heat, that’s why stuff breaks quicker when not properly lubed. Another way that entropy bites us is overheating a blade at the grinder after hardening. Once that blade is hardened, the edge cannot be allowed to heat past the temper temperature. If it does, you have to re-harden, grind that part off or start over because the over-tempered part of the blade won’t hold an edge properly. Entropy is in play because as we raise the temperature of the steel, we release some of the potential change in the crystal structure.
The second law of thermodynamics states that the sum of entropies in a system must always increase, therefore the universe is always moving to higher entropy and therefore more disorder. Any given system can be more or less entropically favorable which means that although the overall level of entropy is always increasing, disorder can happen much more slowly or not at all in certain instances. This depends on the interaction of the energy, entropy and temperature in a system. Entropy actually explains why heat moves from hot to cold - the heat will be more disordered if more dispersed so it will tend to move in that direction.
Although we are constantly fighting it, we use entropy in knife making. Heat treating blades could also be called “entropic manipulation”. I am going to need to lay out the basics of steel heat treating for the must-be-nicers real quick here. The hardness and toughness of steel can be manipulated through temperature cycles. We can Anneal, Normalize, Harden and Temper steel. Annealing is the process of cooling steel slowly to convert it to the softest and most machinable state. Some people leave steel in the forge and let the steel cool slowly as the forge cools, a kiln can be programmed to cool from critical temperature at a slow rate. Normalizing is the process of refining the steel’s grain structure by heating to critical temperature and air cooling a few times with descending temperatures. Hardening is when you get it hot and stick it in the oil. Tempering is when you put it in the toaster oven for a few hours to let the stress out of the blade.
As you can see, we use heat to change the crystal structure of the steel. Not molecules - crystals. If someone uses the word molecule to describe the makeup of steel, they are not speaking from a basic understanding of how steel is composed, don’t listen to them! But I digress. The crystal structures have names. Most annealed steel is in a state called Ferrite, when steel is at or above its critical temperature it is in the Austenite form and the hardened state is named Martensite. When steel is cool, it’s structure is stable and exists in the state that it was last shifted to depending on the heat cycle. We use entropy by applying heat to break down the existing structure which releases the potential for different states. Too much heat/entropy and you get a degraded and overly chaotic structure, not enough and you can’t unlock a steel’s full potential. Many stainless steels, for example, have very stable high alloy structures. They take higher temperatures and longer soak times to break down fully, so if they are not hot enough for too short of a time, the steel will not fully transform to its hardest state.
Tempering on the other hand uses just a smidge of entropy to subtly shift the fully hardened but brittle steel to a good compromise of hardness and toughness. Temper temperature ranges typically from 350°F to 400°F as opposed to a typical high carbon steel’s critical temperature of around 1500°F. As you can imagine, much more dynamic changes take place at the higher temperature. With tempering, we don’t want to change the steel too much, just a bit to take the stress out by allowing any Austenite left over from the hardening process to convert to Martensite. I suspect that the much lower temperature is the reason why tempering takes hours when hardening can be done with a 5 to 20 minute soak depending on the alloy.
I have avoided going into the full conversation about heat treating because there are much better resources, my favorite is Knife Engineering: Steel, Heat Treating and Geometry by Dr Larrin Thomas. There he takes us on the most accessible possible deep dive into the crystal structures (Ferrite, Austenite, Martensite, etc.), grain growth and refinement, alloy effects and really way more than most of us will be able to grasp.
I like to use a baking metaphor for heat treating. In baking a cake, the main components are ingredients, mixing, time and temperature. The alloying elements can be seen as ingredients. Think of flour, sugar and cocoa powder, these are ingredients that give a cake its main flavor and texture properties. Then there are ingredients like baking powder and soda, salt, oil, milk or eggs which act as binders or leavening agents. We don’t want to taste baking powder but we need it in order for the cake to rise to its fluffy texture. In the same way the iron, carbon and chromium (when present) are elements that give steel some obvious properties but Vanadium, Manganese and Molybdenum are elements that modify the other elements in the heat treating process. Manganese can help draw the hardening reaction through the steel evenly, just like baking powder can produce a chemical reaction throughout a cake. Different alloys of steel have time and temperature requirements just like cake recipes and cooling rates can be critical with both. And of course when you’re finished you can use one to cut the other.
Entropy at the forge
When forging we are releasing the potential energy in our gas or coal to heat the steel. Think about how complex this is. We use the chemical reaction of combustion to release energy from the fuel into the surrounding atmosphere. A small fraction of that heat is absorbed by the steel, creating a semi plastic state that can be more easily manipulated. We then use kinetic energy created by our body which is powered by food to rearrange the shape of the steel - transferring the force from our muscles to the tooling, through the workpiece into the anvil and floor. As we work on the knife, heat is constantly being transferred to the tongs, hammer and anvil face, our own bodies absorb the heat we feel from the forge and workpiece. As we forge a piece of steel, the high temperature accelerates oxidation, rapidly producing and shedding layers of oxides which fall to the floor in the form of black forge scale which add to the baffling amount of detritus we sweep up later.
We create a high entropy environment that is highly inefficient but allows for the greatest amount of transformation. A stock removal system where we use a reductive process to shape metal is much more efficient and controllable but forging can stretch and compress steel in infinitely malleable possibilities. We also find that as entropy increases, we approach the material’s mechanical limits so that forging brings us closer to the point of disaster. The more heat we use, the easier the steel is to forge but the closer we are to the steel crumbling (called a “red short”) or otherwise burning up because like our cake metaphor, too much time or temperature can ruin a good recipe.
Let’s look at forge welding in this context. This is the forging process that uses the highest heat levels, just above 2000°F. In order for something new to be created, we have to take it to the edge of destruction. Just over 2300°F will begin doing irreparable damage to the steel structure. A forge weld is a diffusion weld in that the heat is spread evenly throughout the piece while the components are being forced together. As the atoms move more rapidly and the crystal structure expands to austenite, the grain structure grows very large. Once we compress the steel layers with the hammer or press, the grains can grow to interlock and as we further compress and process the steel, the layers homogenize.
Carbon, for instance, tends to migrate between layers so that if damascus is made from two steels with different carbon content, the finished product will have a single carbon content which is the average of the two steel alloys in the piece. Other alloying elements like nickel stay in place, that’s how we can still see the layer pattern. This is because carbon is small enough to flow through the crystal structure while the other alloys are large enough to displace iron atoms in the matrix so they are locked in place. Here again, like heat treating, we use the steel’s entropic potential. Instead of allowing the steel to degrade into disorder by overheating without any control, we use the breaking down of its stability to produce the desired transformation.
I think about entropy in another way. There is only one way to make each knife that we produce. Custom knives are obviously unique but even if we try to make a large set of identical knives, there will technically be something slightly different about each one. Imperceptible perhaps but each one was made by a human and therefore some irregularity will be present. On the contrary, there are infinite ways to destroy the knife. Only one way for success and infinite ways to fail. And destruction is easy. Building the most advanced knife in the world may take a lifetime to make but just drop it on a campfire and walk away and it's gone. This may be why many people fall into criticism so easily, it is just so much easier to tear down than to build.
Even without intentionally destroying a knife, oxidation and wear on the edge are good examples of entropy at work. With high carbon steel, just a small amount of moisture can cause rust and that can be caused by condensation due to temperature changes. If you haven’t ever taken a knife out of the sheath after storing it to find a fine layer of rust, just wait, it will happen eventually. Oxygen is funny stuff, we need it to live but it is also the most destructive element on planet earth.
I know this section is getting pretty dense but taking a deep dive into how things work at the fundamental level can inform our view of the grand scheme. Take the atomic bonds occurring in steel. Metallic bonds are a type of atomic bond. Iron ions are atoms which have shared or lost one of their valence (or outer) electrons which leaves them with a positive charge. If all the iron atoms in a crystal were positive with no other influence they would fly apart but the atoms are arranged in such a compact structure that the valence electrons are floating free between the atom nuclei and take on a negative charge. This is the “Electron Sea” model and explains the conductivity, ductility and malleability of metals. Because the electrons making the bonds are not rigidly locked in place, it allows for the crystals to shift without breaking apart and the electrons can freely move along the boundaries to conduct electricity. Pretty cool!
Getting a basic grasp of how things are held together can really help us understand how to work with the material and also understand why it fails sometimes. Take the example of breaking off the tab of an aluminum can: bend the tab back and forth a few times and the aluminum breaks. Why? Each time we bend a metal, we work-harden the piece just a bit. The movement slides the crystals against each other until they lock together in a way that allows no further movement. As the piece hardens, it becomes brittle until there is no room in the grain structure for further ductility and BAM! - the piece snaps. Where we once had a nice even atomic lattice with a sea of electrons judiciously shared out, we now have a compressed and chaotic mess ending in mechanical failure. This also explains why knives are so hard to straighten cold, the bent section is slightly harder from the previous bend and therefor resists bending while new areas more readily absorb the force of the new bend - resulting in cussing, or throwing the knife on the ground and jumping up and down on it. Usually both.
The coarse interior texture that you see on the broken end of a piece of metal is the grain. When the metal cools from its critical temperature or from its molten state, crystals begin to form and align into grains. We can manipulate the grain size just as we manipulate the crystal structure itself. Alloy elements like Vanadium affect grain size but the heat treat process is a much more potent tool. This is the normalization process and is performed between forging and hardening. See, forging a blade is really disruptive to the steel structure. While forging, we are not only intensely deforming the steel from its original state but were also overheating the piece as well. Stretching and compressing the steel stresses it out but overheating really messes up the grain. The larger the grains grow, the more brittle steel becomes, and after forging, we have a piece of steel that is stressed out and has large and uneven grains.
At this point our steel needs some self care time. Just some time in the sauna to relax. If we give our steel some time to live, laugh and love then it will come back to work refreshed. So we pop it in the kiln or forge until it is heated to the top of that steel’s heat treat range. For most high carbons this is around 1600°F. The steel is allowed to air cool and the grains are refined a bit. Most high carbon steel benefits from heat cycles at 1600°, 1500° and 1450°. The middle temp is kind of arbitrary, it just represents a value between the highest and lowest. When this steel relaxation process is finished, you can actually see the difference in the grain. It’s pretty cool to see with your naked eye the difference in the atomic structure of your knife. Of course you have to break it to get a look but you know what they say “If you want to make an omelet, you have to break some knives.”
Here’s why we do all that extra work to refine grain: if you have two knives made in exactly the same shape and heat treated and tempered to the same hardness, the knife that did not have its grain refined by normalization will be weaker and more brittle. Some makers insist that a coarse grained steel cannot become as sharp as a fine grain steel - which makes sense to me. So when you are considering buying a hand forged blade, you definitely want to ask about the maker's heat treat process because the way they do or don’t program the atomic structure of the steel will determine if the blade performs well or not.
There is an interesting piece of blacksmith lore connected to normalizing. “Packing the edge” is a technique that is still used by traditional smiths. This is the process of planishing (lightly tapping) the edge of the blade to compress the steel along the cutting surface. This is done with the spine against the anvil and the hammer striking the cutting edge. This is nonsense - but it works! What’s going on here is that smiths that packed the edges of their blades tended to produce better blades. It makes intuitive sense to compress the steel along the cutting edge so that it is more dense and therefore harder or sharper or something. What is really happening that makes the knife better is - you guessed it - normalization! If you take a knife in and out of the forge a few times at or slightly below critical temperature and only lightly planish without heavy forging, you allow the crystal structure to refine. Voilà! You have a better knife than the other guy who doesn’t “pack his edge.” I have to admit to some edge packing from time to time myself, but that’s just because the edge gets lumpy from forging in the bevel, and it gets lonely in the forge sometimes.